
Whether you are a brand-new paramedic or nurse or a seasoned critical care provider, chances are you have started or maintained a patient on vasopressors. They are considered to be one of the hallmark drugs of resuscitation and what we consider to be “life support” drugs. There are a few key drugs on the market that have fallen into/out of favor over the years for various reasons but there remains a significant amount of controversy on what the “best” agent is to use in a given situation.
In this article, we step out of the clinical context of what the preferred vasopressor is for a given clinical situation, but instead take a look at the scientific concepts underlying their administration and efficacy as key players in resuscitation. Specifically, we will look at those vasopressors derived from tyrosine and phenylalanine, though many of the biological concepts presented here extend to many other drugs as well. It is my hope that by reading on you gain a better understanding of these agents to better apply them in your clinical practice, and that you learn or refresh your knowledge of biology and chemistry along the way.
Let’s dive in…
The Making of a Vasopressor

Above is what looks like a complicated synthesis pathway, a dreaded topic among many students in organic chemistry courses. In this context, our goal is just to understand how our vasopressors are made in the body, and we can break down this complex pathway into more digestible components.
First, looking at the picture above we can see that the process of forming the vasopressors: dopamine, noradrenaline (norepinephrine), and adrenaline (epinephrine).
Phenylalanine and tyrosine are both amino acids that are considered to be a part of the 20 essential amino acids the body requires to carry out many of our necessary reactions, and as the building blocks for other compounds (like nerdy legos).
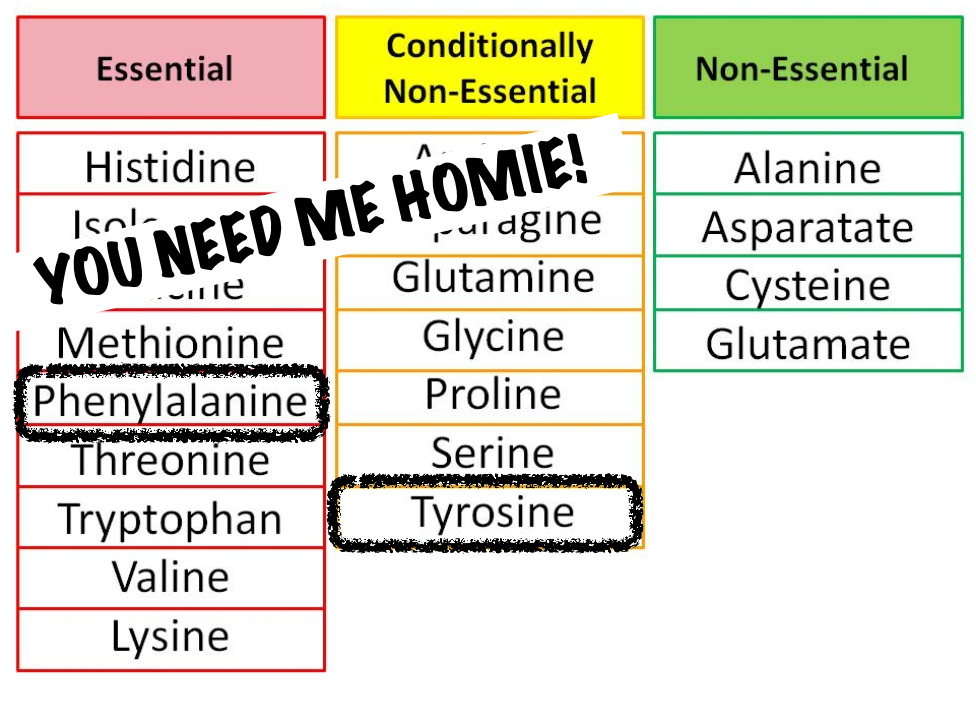
Both tyrosine and phenylalanine fall into the class of amino acids referred to as aromatics (literally because these enzymes have a sweet odor..). Let’s talk a little bit of painless organic chemistry and the concept of an aromatic structure.
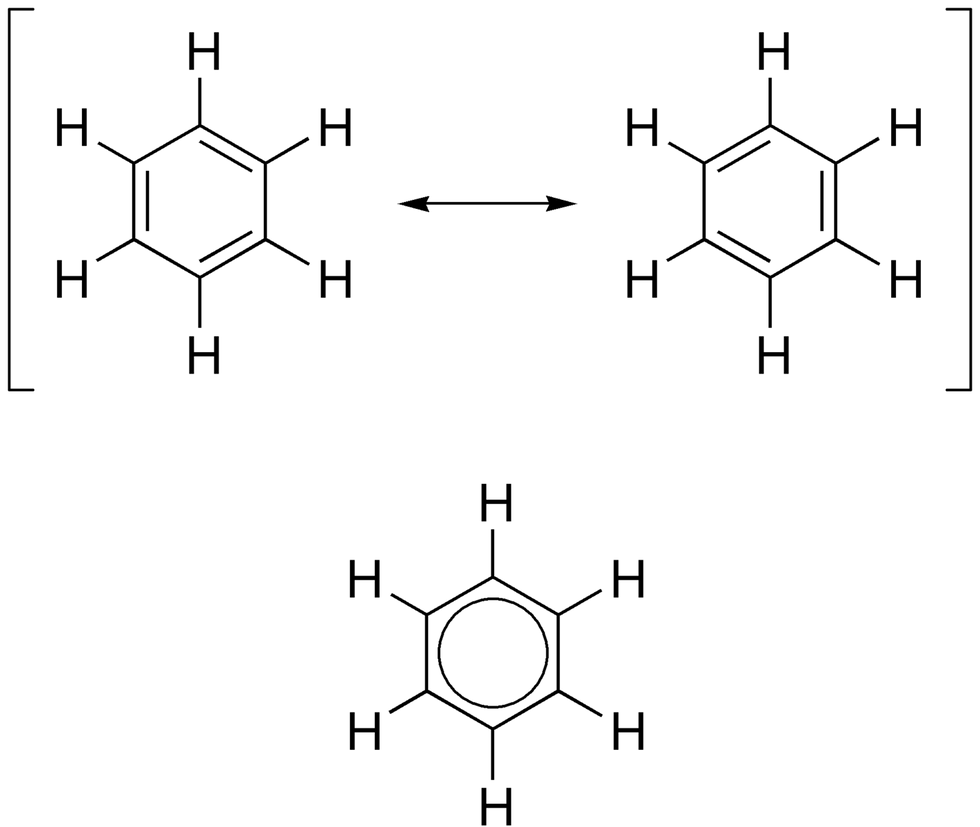
Aromatic ring structures are considered to be a very versatile form of an organic molecule. One of the most common structures is benzene, an aromatic ring of six carbons forming a hexagon. The intersecting lines of the hexagons above each represent a carbon.
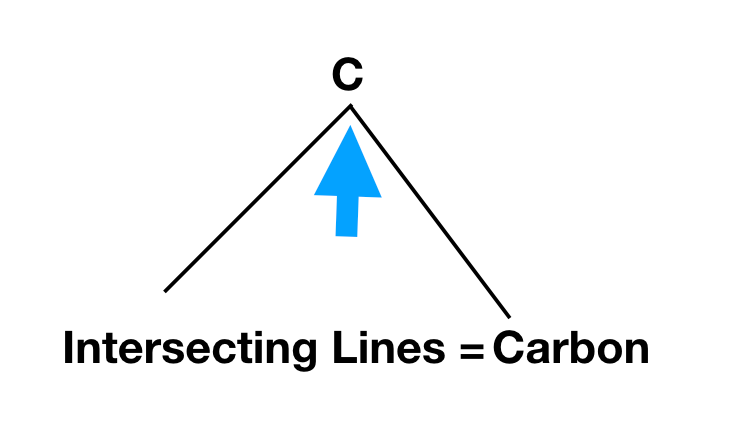
Anytime you observe a second line, it indicates that there are two bonds attaching the carbons. On most organic structures you will not see hydrogens, but they exist to satisfy a total of 4 bonds made by each carbon. In this case, there is 1 additional H assumed to be attached to each carbon.
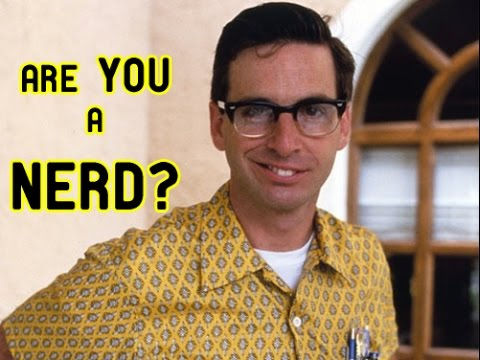
Why do we care so much about this?
It is a basic building block for adding things and moving other groups around, or forming new compounds. This is what makes these aromatic rings so versatile. Ok, now let’s address how they apply to our vasopressors above.
If we start at tyrosine and we want to get to the compound L-Dopa, we need the compound tyrosine hydroxylase (the finger below) to make that happen. If we break down the words, anything that contains “-ase” is an enzyme, and hydroxyl groups are the -OH groups you see on many of the molecules above. Legos don't build themselves. You need an enzyme to stack those puppies up!

The basic concept here, to go from tyrosine to L-Dopa we need to add a hydroxyl group (an -OH) to tyrosine. Once that happens the new compound has different biological characteristics than the previous compound. Because of the chemistry of the -OH group on L-Dopa it can now bind receptors differently and it reacts differently at physiological conditions inside the body.
For example, Parkinson’s patients are often administered L-Dopa as part of their treatment regimen to replace needed dopamine in the brain. L-Dopa can cross the blood brain barrier more readily, so it is administered in the L-Dopa form and converted to dopamine once inside the brain. Another important point to consider is what we consider “vasopressors” derived from tyrosine, are also neurotransmitters that function within the brain carrying out other functions.
Back to the chemistry… to get from L-Dopa to dopamine, we need to do some rearrangement, and, in the process, we get rid of carbon dioxide. There is a loss of 1 carbon molecule and 2 oxygen molecules and we move the NH2group, called an amino group, to the end of the molecule. Again, this rearrangement changes how this molecule will interact at different pH levels, with different receptors, and with different membranes. The molecules are very similar, but the groups attached to them (NH2, OH, etc) change their functional abilities.
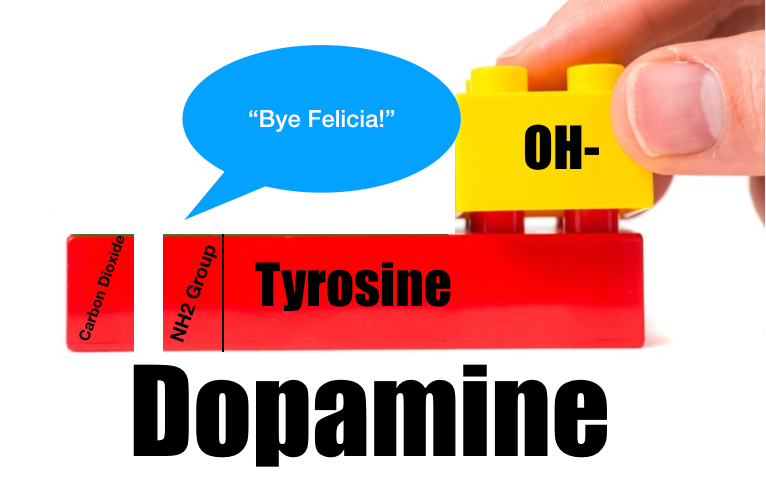
In going from dopamine to noradrenaline (norepinephrine) and from noradrenaline to adrenaline (epinephrine) take a look above to see what’s added and what is re-arranged. Then look at the arrows and see if you can make sense of the names of the enzymes, or reaction intermediates, that were needed to make those changes occur. I would explain them here, but I think it would be more beneficial for you to see for yourself how that change occurs now that you have the education to decipher what just a few minutes ago may have looked extremely complex.
Now that the molecule is made, let’s discuss how they work in Part 2! Stay Tuned..

Tom Latosek, MS, NRP, CCP-C
Tom is a practicing paramedic and EMS educator who is interested in EMS research, and advancing the profession of EMS through education. Tom has practiced in a variety of EMS clinical settings and teaches a variety of courses for a healthcare education company. Tom holds an MS in neuroscience and a bachelor’s degree in biology and psychology and is currently a first-year medical student.