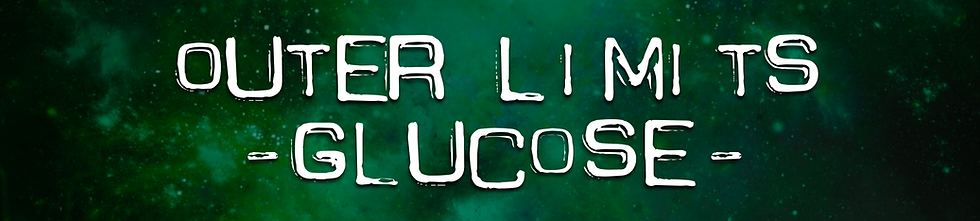
Welcome to the fourth and final installment of this metabolic panel series, where we're exploring what happens when these lab values hit their limits, or beyond. Previously we've explored Cations (located here), Anions (located here), and Renal Values (located here), but don't feel like you need to read these in any particular order.
These blogs are meant as a reference for you to come back to. There's a lot of information in each, so they might be better absorbed in chunks. I'm writing these as the reference I wish I had when I started learning lab values.
This week we're going to take a deep dive into the most commonly measured lab value - our blood glucose. First, we're going to evaluate what glucose is, and how the body keeps it in tight control. Then, we'll look at what happens when glucose hits its limits (and beyond).
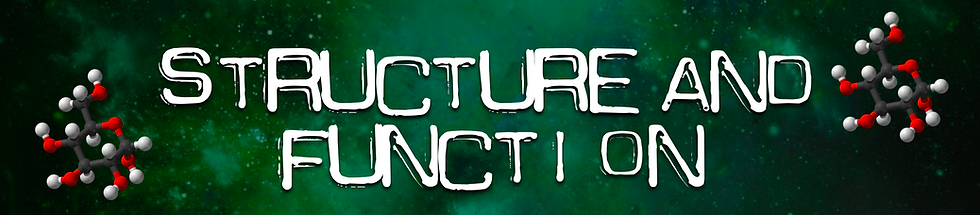
Glucose is a 6-carbon molecule, a monosaccharide. We generally get glucose either from directly ingesting it or from breaking down long chains of sugar (disaccharides or polysaccharides) that we store, breaking down protein, or fat. The length of the sugar chain determines how long it takes for the body to break it down. The longer a chain of sugars is, the more slowly your blood sugar spikes. The shorter a sugar chain, like a disaccharide, the faster the blood sugar spikes. A monosaccharide is immediately available since it doesn't have a 'chain' that needs to be broken down.
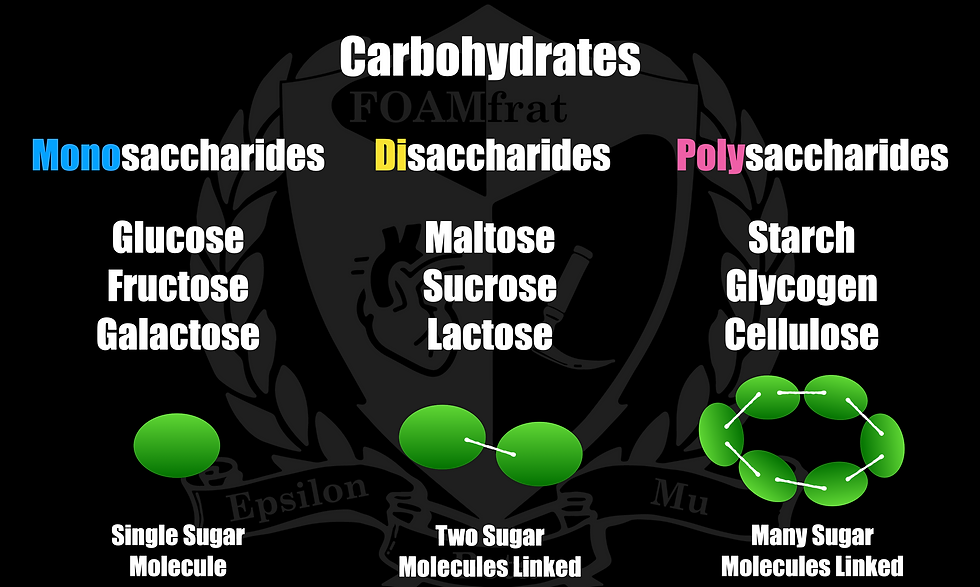
We all know that this glucose is a very usable form of energy (as long as we have insulin to get it into certain cells). However, what if we didn't intake any carbohydrates in our diet? This is actually a pretty interesting part of nutrition. The body can survive without carbohydrates - it's not a requirement for the body to function (some argue that fiber is needed, others disagree). But wait, if we're not taking in any carbohydrates, won't our blood sugar drop to nothing? No - that's where a process called gluconeogenesis comes into play. Gluconeogenesis converts non-carbohydrates such as fatty acids, lactate, and amino acids into glucose. Essentially, as long as we have fat and protein, we have the ability to make glucose (fat and protein are essential to survival) (1). As a side note, no, that doesn't mean you start losing a bunch of muscle by not eating. This is why individuals who eat a strict ketogenic diet do not become hypoglycemic. There are even carnivore diets, out there, in which someone eats only meat and their blood glucose never drops. This is, of course, a generalization and oversimplification. We know that there are individuals who have metabolic derangements that cause blood sugar to drop regardless of what they eat. We also must remember the diabetic population, who take medications to lower blood sugar, can put them in a hypoglycemic state sometimes regardless of the presence of carbohydrates or protein in their system. These diabetic patients, however, initially present with hyperglycemia, and will usually only have 'lows' once the medication is introduced.
We'll talk a little about what the body does to regulate glucose, and then we'll move on to lots of clinical applications.

Glucose in our blood is not the same thing that ends up getting used to create energy (ATP). It's the starting point, but a lot of things have to happen to it first. Glucose gets chopped up, rearranged, and has phosphates coming and going all before it's converted into pyruvate (an alpha-keto acid) that enters the Krebs cycle when oxygen is present. Glycolysis will occur regardless of the presence or absence of oxygen, yet oxygen is required for pyruvate to enter into the Krebs cycle. The actual process of chopping up glucose does give us a little bit of ATP, though. We get a net of 2 ATP just from the process of glycolysis (we make 4 ATP total but we had to invest 2 ATP at the beginning of glycolysis to get things rolling) (2).
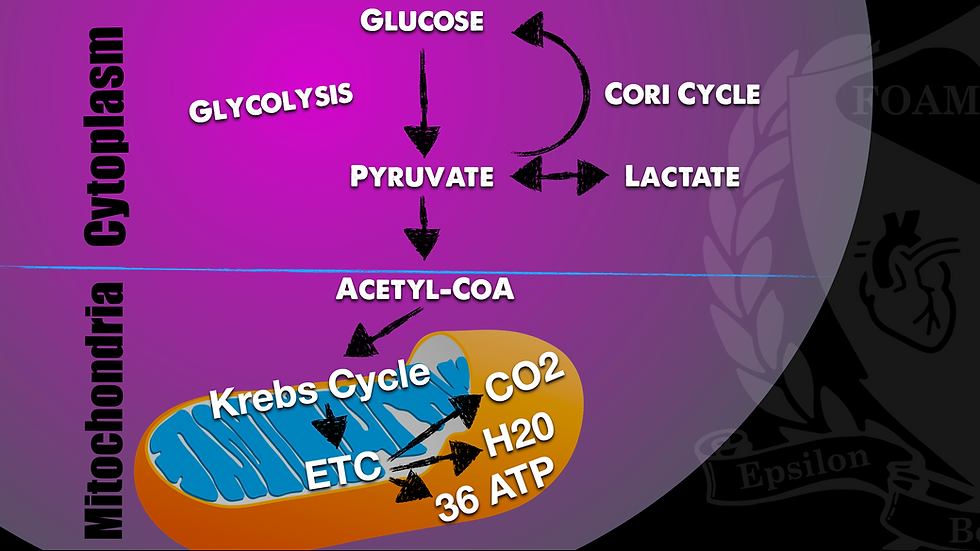
Once glucose starts the process of glycolysis, which happens inside the cytoplasm of the cell, it's no longer blood glucose because it's not in the blood (serum) anymore. The glucose is now chopped up/lysed into what will eventually be pyruvate. How is this process regulated? I'm going to briefly focus on the hormonal regulation of glycolysis, but there is a lot of allosteric regulation as well. Allosteric regulation is very interesting, but I'm not going to go over all of its mechanisms here.
Hormonal Regulation
Insulin and glucagon are our regulatory hormones of glycolysis. Insulin promotes glucose breakdown, while glucagon inhibits it. Both of these hormones are released by the pancreas in response to blood glucose levels (3). Let's talk about what happens when blood sugar is high, and then what happens when it's low.
This illustration will make a lot more sense after you read the paragraphs below.
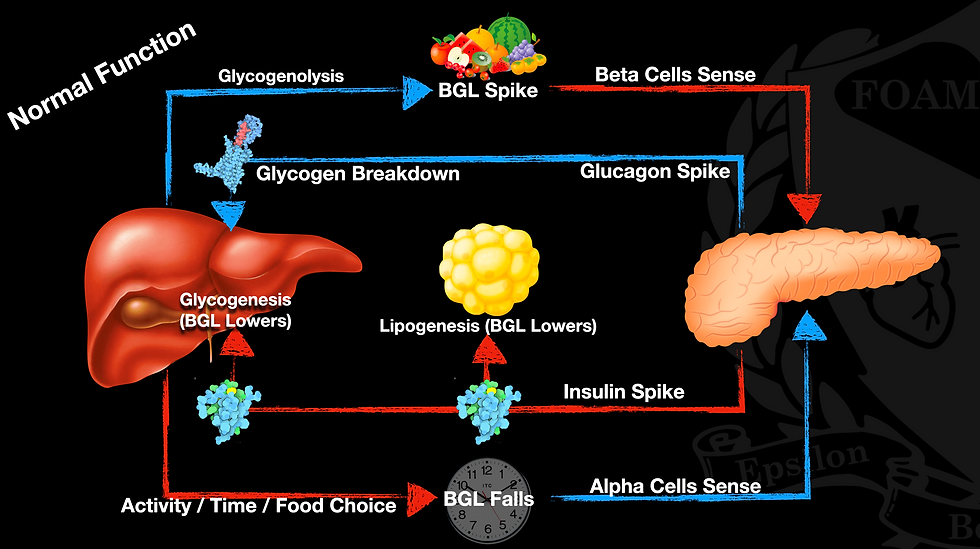
When blood sugar is high, the body recognizes this excess and wants to store glucose for later use. So, what does it do? As the pancreas senses this increased glucose level, its beta cells respond by releasing insulin into the bloodstream. Insulin is an anabolic hormone... anabolic? An anabolic process is one in which we take a simple molecule and make it into a more complex molecule. Why would we turn glucose into a more complex molecule? We do this in order to store the glucose. We can do this in a couple of ways. First, we can store the glucose as glycogen, which is a pretty long-chain polysaccharide consisting of 24 carbons (that's 4x more than glucose). This glycogen gets stored in the liver, and a little bit in the muscles, as well. Second, insulin lets us store glucose as long-chain fatty acids in fat (adipose) tissue after a conversion process. What about when the blood sugar is low?
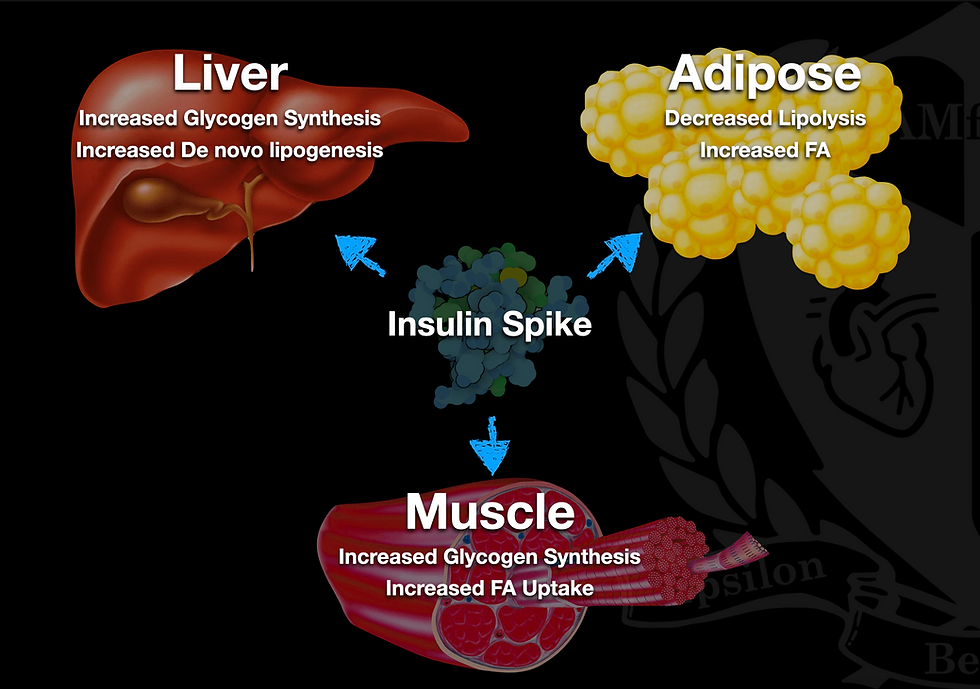
When blood sugar is low, the pancreas recognizes this state and releases glucagon from its alpha cells. Glucagon causes the liver to undergo a process called glycogenolysis (the lysing of glycogen). Remember glycogen? That long-chain polysaccharide that insulin made and stored in the liver? Glucagon breaks that down by chopping it up into glucose. Glucagon also increases lipolysis (fat breakdown). This process is considered catabolic - taking complex molecules and making them more simple (and in this case, usable). Glucagon is considered to be the chief catabolic hormone of our systems. As a side note, from hormonal glucose regulation, it's worth mentioning that endogenous epinephrine also plays a key role in the response to hypoglycemia:
"Epinephrine increases glycogenolysis and gluconeogenesis at the liver; reduces insulin secretion while increasing glucagon release from the pancreatic islets; reduces glucose uptake and utilization and increases glycolysis by muscle, and increases lipolysis in adipose tissue." (4)
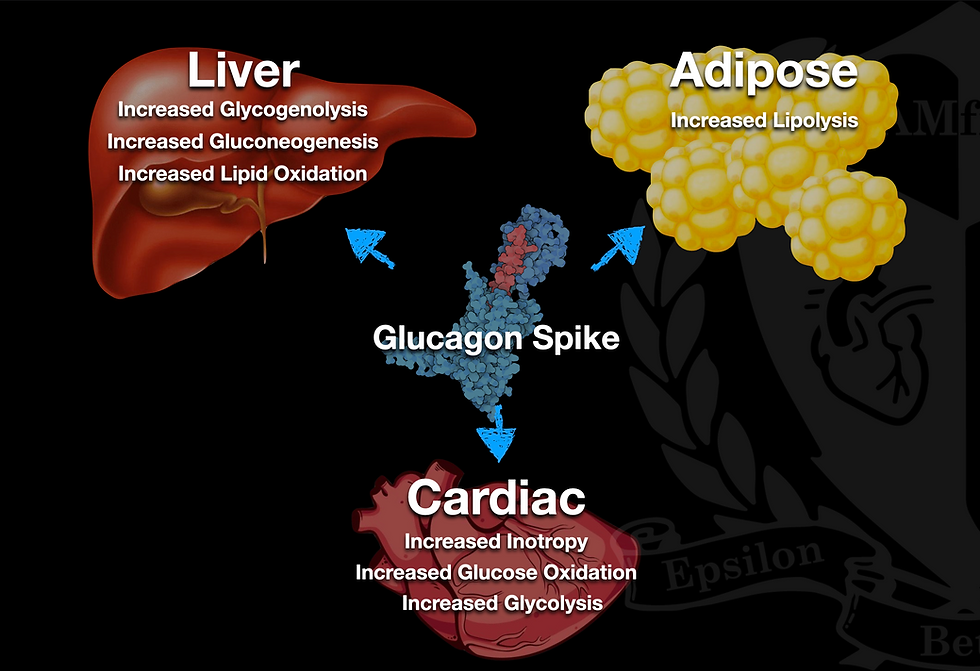
When we administer either insulin or glucagon, we're essentially attempting to act as a normally functioning pancreas in the absence of one inside of the patient.
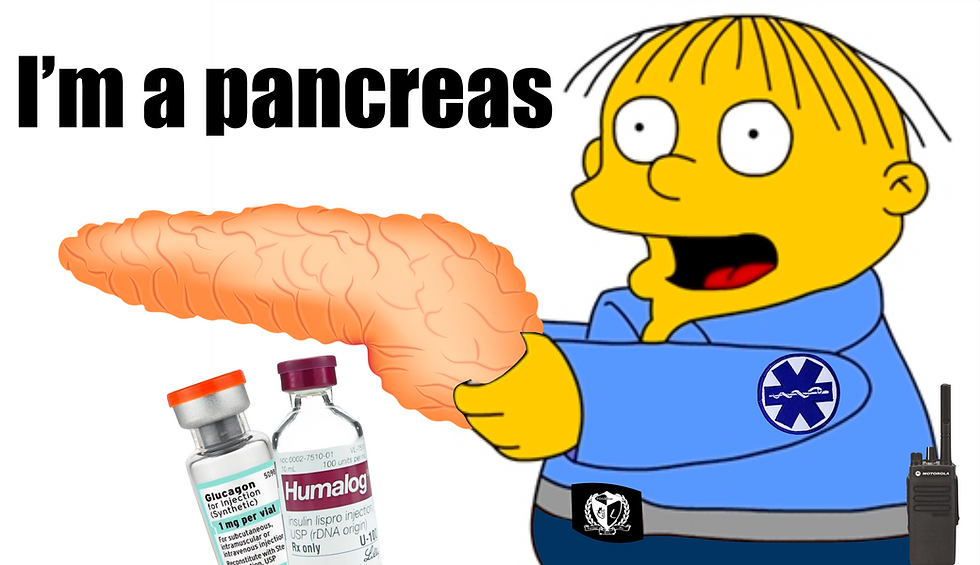
Exogenous Insulin and Glucagon profiles.
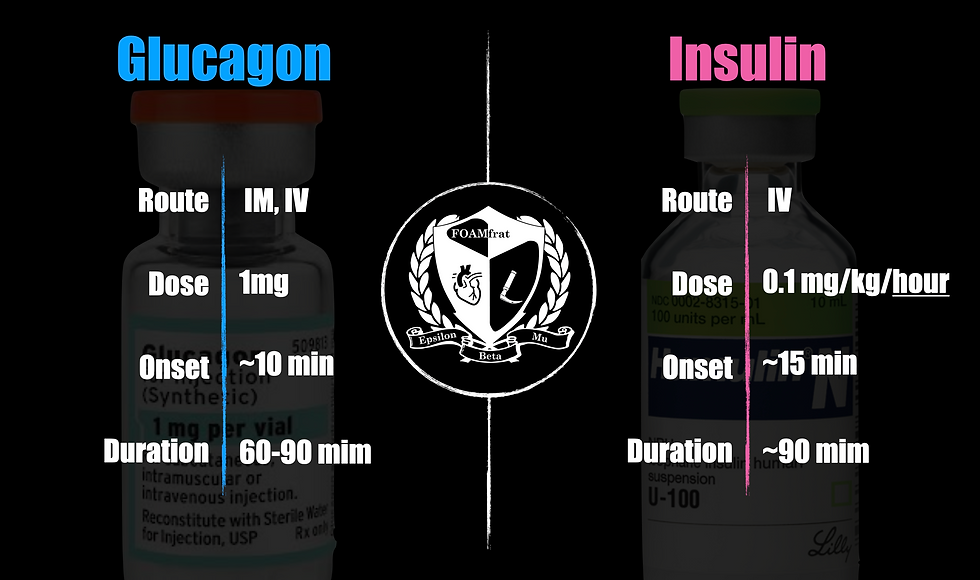

No matter what you eat, you always need glucose in your bloodstream. Even those who do not eat carbohydrates/sugar, always have enough fat and/or protein to break down, in order to make blood glucose. This goes for those whose energy is primarily coming from ketones as well - they still should have normal blood glucose readings (albeit probably on the lower end of normal). We all recognize the signs and symptoms of hypoglycemia - sweating, dizziness, tremors, tachycardia, altered mental status, and even altered level of consciousness when it becomes very low. It's no wonder why a hypoglycemic patient can get confused for someone who is under the influence of drugs such as alcohol or the like. Why do patients get these symptoms? It comes back to what we just discovered about epinephrine a moment ago.
Hypoglycemia sends the body into a state where it's desperate for energy. The signs and symptoms associated with this low glucose state seem to follow a familiar pattern - sympathetic (SNS) and parasympathetic (PSNS) overdrive. Wait, both? Our organ systems are constantly in a state of balance between SNS and PSNS influences. In hypoglycemia, each portion of the nervous system is in some way acting in an elevated way, just in different places. How so?
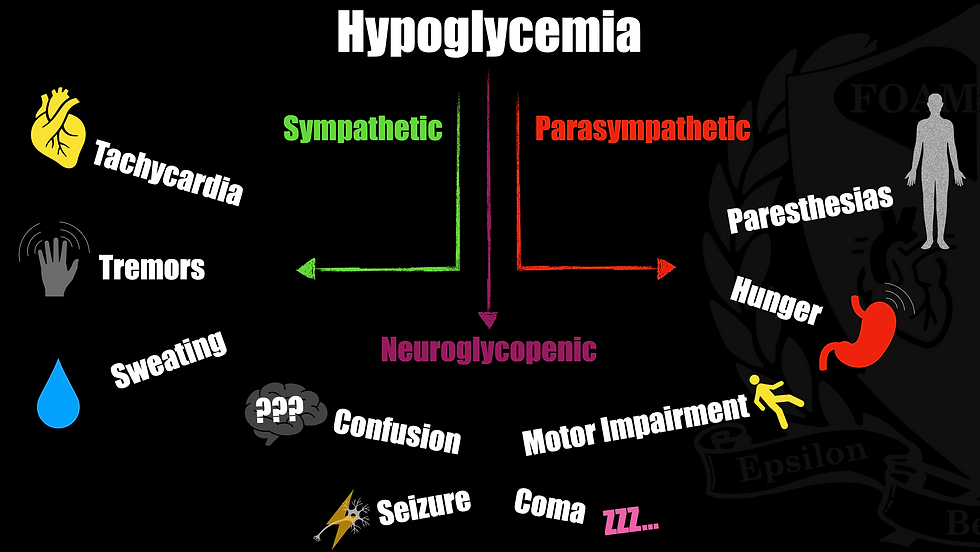
The SNS is making the patient experience things like tremors and tachycardia. However, the PSNS is making the patient hungry and experience paresthesias. This is the body's attempt to keep the patient going in this time of low glucose availability (4). However, as glucose becomes too low, encephalopathy occurs. A condition called neuroglycopenia creates an altered mental state. Neuroglycopenia is low blood glucose levels in the brain, which is the result of low serum blood glucose. Neuroglycopenia is what accounts for the neurological issues we see with hypoglycemia. In reading this about neuroglycopenia, you might conclude that the only way you can get neurological symptoms from hypoglycemia is if the serum blood glucose is low - but that's actually not true. You can experience low brain glucose levels in the absence of systemic hypoglycemia. How is this possible???
In a patient with neurological symptoms and normal serum blood glucose (115 mg/dL), check out the treatment chosen in this 2020 case report. See if you can fill in the blanks before the following paragraph!
"Intravenous dextrose was administered, as utilization of glucose by the central nervous system (CNS) is increased during __________, and serum glucose concentrations may not reflect those in the CNS. Damage to the CNS via neuroglycopenia can occur at normal serum glucose concentrations, making dextrose supplementation a standard treatment for ____________." (5)
...?
Salicylate toxicity! In salicylate poisoning, the local neurological glucose usage is very high but not so high that it drains the whole system and causes generalized hypoglycemia. It's just enough to subtly starve the brain of energy and cause neurological damage while you and I believe glucose levels are not the problem.
There are other causes of neuroglycopenia in the absence of systemic hypoglycemia as well, but it is not possible to cover them all. A condition called hypoglycorrhachia (low glucose in the cerebral spinal fluid) can occur from disease processes like meningitis (multiple types), leukemia, cancer (tumors), and GLUT-1 receptor deficiency (and quite a few others) (6). However, you would need to test CSF glucose (normal level should be above 2/3rds of a normal serum glucose level) in order to detect these. The reason why the CSF glucose levels can be abnormally low is that these, cells that aren't normally there (ie. bacteria, fungus, leukocytes, tumor cells, etc.) metabolize the glucose locally, thus leaving too little for the brain.
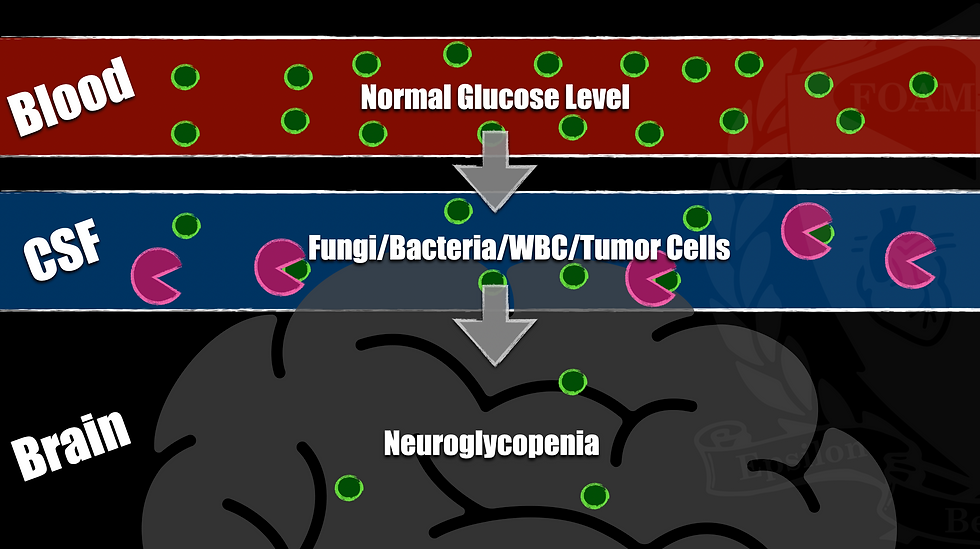
When we think of the typical case of systemic hypoglycemia, the majority of these cases are mismanagement of diabetic medications, commonly insulin. However, there are cases that occur in people without diabetes, as well, but they are rare. Some causes of hypoglycemia in the the non-diabetic patient include a tumor in the pancreas that secretes insulin (insulinoma), non-islet cell tumor hypoglycemia, or even factitious hypoglycemia. For the rest of non-diabetic patients who present with hypoglycemia, it's usually a mix of factors, such as infection, alcoholism, starvation/exhaustion, or multiple organ failure. (7)
With these cases being pretty uncommon in non-diabetic patients, the majority of the hypoglycemia we will encounter is rather specific - a diabetic patient who is having a medication issue. So, what is the current best practice for reversing hypoglycemia?
If the patient is symptomatic but is protecting their airway, following commands, and generally seems like a good candidate for oral intake, we should be giving them fast-acting carbohydrates (monosaccharides) (7).
Administer ~20 grams of simple carbohydrates (examples below).
Monitor for at least 15 minutes.
Re-check blood glucose.
70 mg/dL or below? Repeat step 1.
Give them a snack with complex carbohydrates.
Simple carbs? This would include oral glucose (tube/tabs), fruit juice, crackers, honey, or even candy. Complex carbs would include whole grains or certain fruits. Whatever you're using, it's usually very easy to figure out how many grams of sugar/simple carbs or complex carbs are contained in it.

What if they're not a candidate for oral glucose replacement?
If you don't have an IV, or you're waiting for one, administer 1mg of glucagon IM. But, don't expect any miracles, since it could take up to 15 or 20 minutes to work. If it does work, you should probably feed them pretty quickly because they are going to crash again (and probably have emesis).
If you have an IV, obviously dextrose (dextrose is just a different form of glucose) is the way to go. However, how you administer this dextrose is the cause of some debate. I'll tell you what I believe is the best and safest way to administer IV Dextrose.
Start an IV and hang a 250mL bag of 0.9% NS.
Infuse ~50mL and check the site carefully for and extravasation (only NS at this point).
Inject an entire amp of D50 into the bag of 0.9% NS (the bag is now essentially D10).
Administer 100mL to the adult patient, or 2mL/kg to the pediatric patient, and reassess.
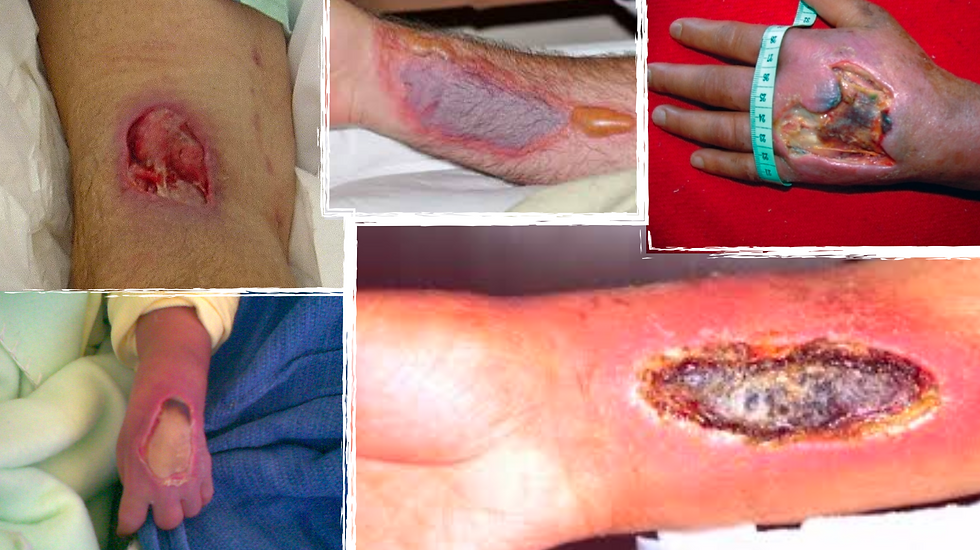
We all know the dangers of extravasation with high concentration glucose. Gone are the days of hooking up the D50 amp directly to the hub and pushing as fast as we can. I believe this system above is the safest and most efficient method of administering IV dextrose, but let me know if you can think of a way to make this more efficient or safer! So, can we sign the patient off now?
That's up to you and your service to decide. Personally, I would not sign off a diabetic patient without monitoring them for at least 20 minutes post-correction and ensuring they've eating complex carbs (and preferably some fat and protein as well). However, that's just my opinion. As usual, and for anything you learn here, follow your local guidelines.
Does anyone else remember what Tyler's mom said she would do for him in such an emergency? Rectal Mrs. Butterworth. Probably zero chance for aspiration.
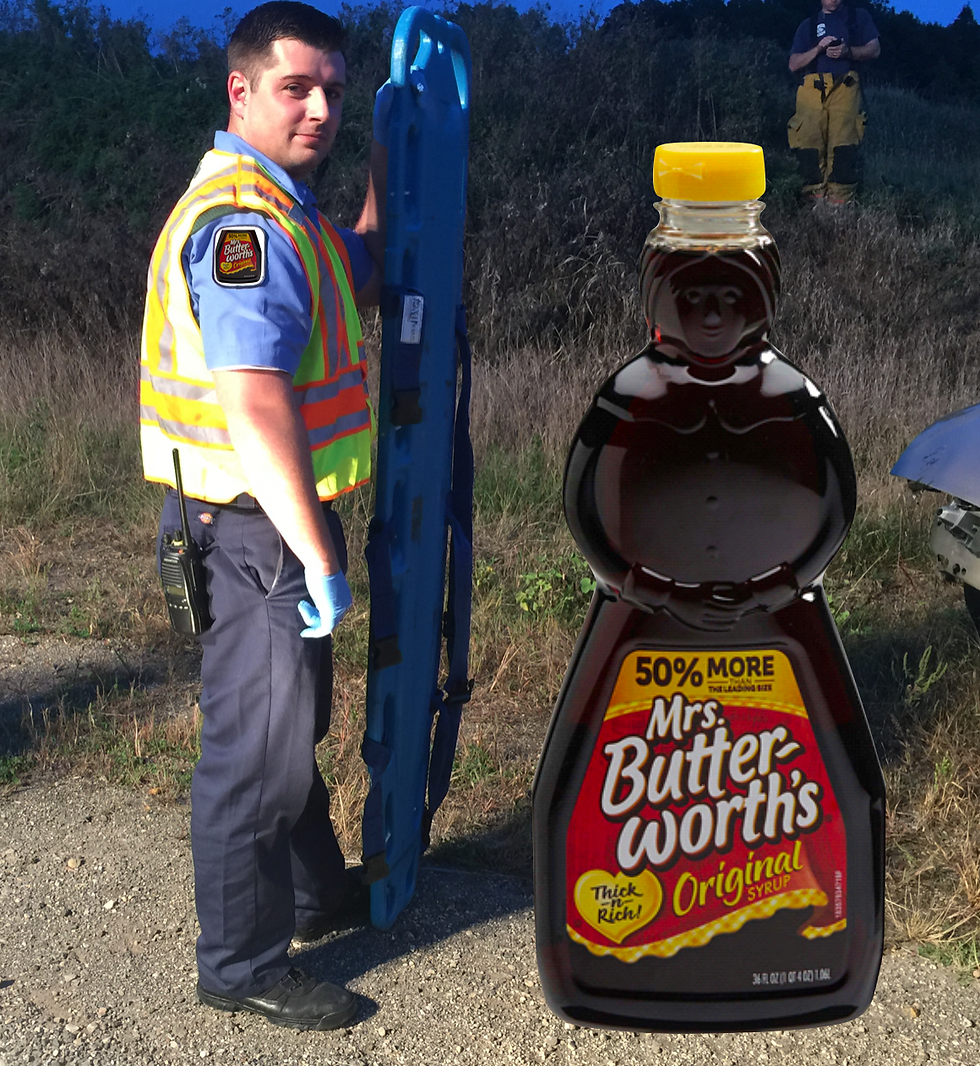
Aaaaand that's probably a good place to leave hypoglycemia...

Severe hyperglycemia (just like severe hypoglycemia) is somewhat rare without the setting of diabetes. A patient can get stress-induced hyperglycemia, but it's seldom as high as what we would see in diabetic ketoacidosis (DKA), and especially the hyperglycemia seen with hyperglycemic hyperosmolar state (HHS). These stress-induced hyperglycemic patients are usually critically ill, and the criteria are thought to be >110 mg/dL (previously >200mg/dL). What causes this stress hyperglycemia?
"A combination of several factors, including the presence of excessive counterregulatory hormones such as glucagon, growth hormone, catecholamines, glucocorticoids, and cytokines such as IL-1, IL-6, and TNF-α, combined with exogenous administration of catecholamines, dextrose, and nutritional support, together with relative insulin deficiency plays an important role... Increased gluconeogenesis and hepatic insulin resistance are the major factors leading to hyperglycemia." (8) The same source indicates that insulin resistance in sepsis plays a key role in sepsis-induced stress hyperglycemia.
There are, of course, lots of medications that induce hyperglycemia to various degrees without the presence of diabetes as well. Some of the most common are steroids, some antipsychotics, and statins. Over-the-counter medications like niacin and pseudoephedrine can raise blood glucose slightly. And medications that we administer with beta stimulation (like epinephrine and albuterol), can raise blood glucose as well.
Stress or medication-induced hyperglycemia are things that we note, and surely in more long-term care they are important findings - prolonged hyperglycemia is likely to be deleterious. However, are we going to treat stress hyperglycemia? Or a slightly raised blood glucose due to medication? It's not very likely. Just like with the hypoglycemia we talked about above, we're going to be much more frequently seeing and treating diabetic patients with more severe hyperglycemia and the sequelae that go with their particular brand of glucose derangement. So, what's so different about diabetic hyperglycemia?
Let's briefly cover the type-1 diabetic first, who gets diabetic ketoacidosis (DKA).
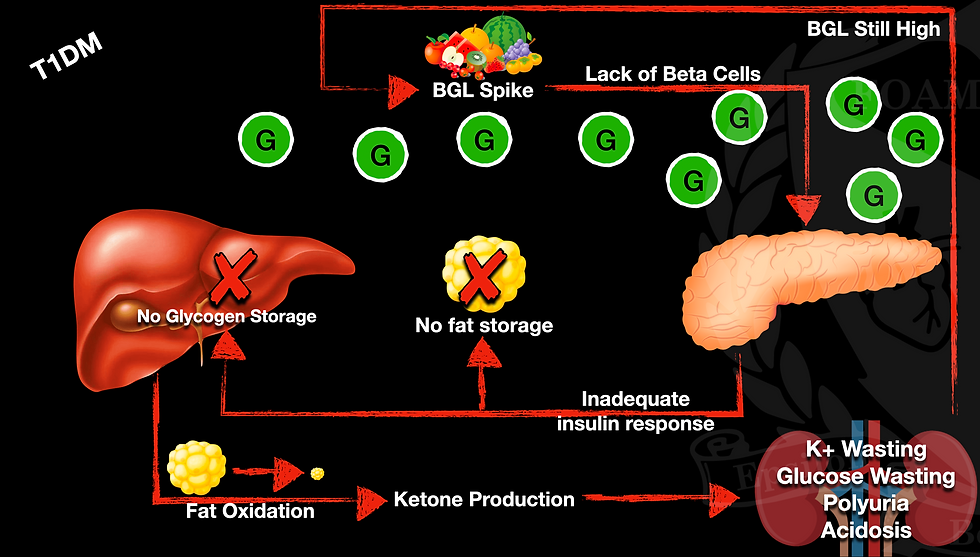
DKA is due to a lack of insulin from the beta cells in the pancreas. Without this insulin, the glucose gets stuck in the serum in high amounts, causing hyperglycemia. The hyperglycemia doesn't actually have to be that bad in DKA for the patient to be very sick. In fact, it doesn't have to be high at all. You can have a euglycemic DKA (DKA with normal blood glucose). Alright, so these patients get hyperglycemic because they don't enough insulin - what's the big deal?
They go into profound anion gap metabolic acidosis due to massive ketone production (ketones from the fat metabolism that they're thrown into).
They start urinating glucose (glucosuria), which water follows, and then a process called solvent drag causes them to lose all kinds of other electrolytes (potassium especially becomes a problem for several reasons).
That urination causes massive dehydration.
Kussmaul respirations also lead to dehydration due to insensible loss, but they need to maintain minute volume to compensate for the metabolic acidosis.
So we have a patient who is very acidic, requires a high minute volume, is massively dehydrated, and has multiple electrolyte abnormalities. It isn't so much the hyperglycemia that is making them so sick, but mainly the altered metabolism. We have a few blogs and podcasts on DKA, so I don't want to get in-depth here. I'm to going to oversimplify the treatments here because you can find the details in these blogs/podcasts:
Essentially, we have to ensure ventilation, replace volume, ensure the potassium is managed appropriately, administer insulin, and lower glucose in a slow and controlled manner (~ 75 mg/dL per hour) to prevent fluid shift and cerebral edema. All of those treatments are in order of operation as well. If you change the order of those treatments, you can really screw things up. (9) What about HHS in the type-2 diabetic?
How is HHS (seen in type-2 diabetics) different from DKA?
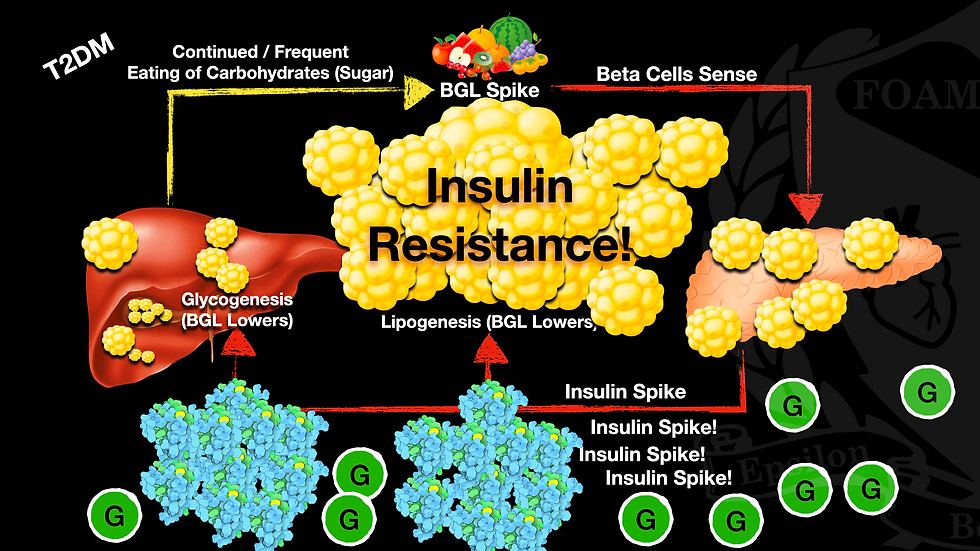
In DKA we had a lack of insulin, which set off all of the events mentioned above. In HHS, we have a totally separate issue - which is that there is an abundance of insulin floating around, but the patient is resistant to that insulin (insulin resistance). We can think about the insulin levels in HHS as relatively low since there is no longer enough to get the job done. There is a lot of discussion and debate about what exactly insulin resistance is, but I tend to believe the theory that insulin resistance is like trying to cram clothes into an already full piece of luggage. The liver, adipose tissue, and muscles, are already at their max capacity for energy storage, and then the body starts to require more and more insulin to stuff more energy (glucose) into those cells. It's like how you can't zipper your luggage by yourself, so you need to get someone to sit on the luggage while you force the zipper. If things get really bad, you might need to enlist even more help.
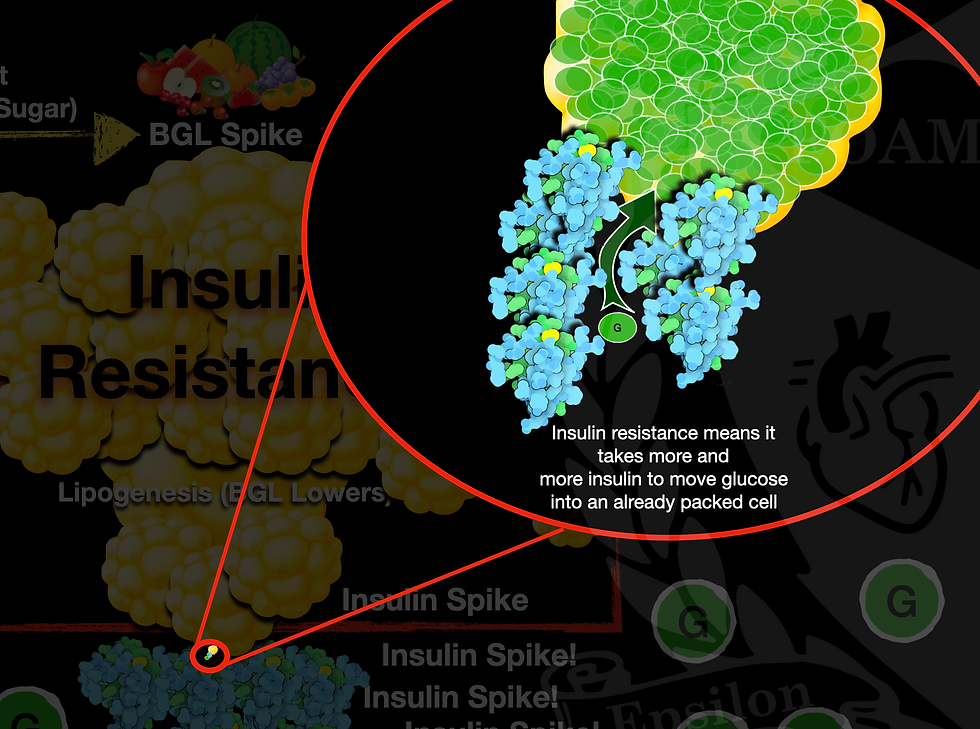
The reason this type of illustration makes sense to me is the difference in body mass index (BMI) in type-1 and type-2 diabetics. Type-1 diabetics have very little insulin, which is an anabolic hormone, so they have a very low BMI. On the other end of the spectrum, type-2 diabetics generally have a high BMI due to high amounts of insulin.
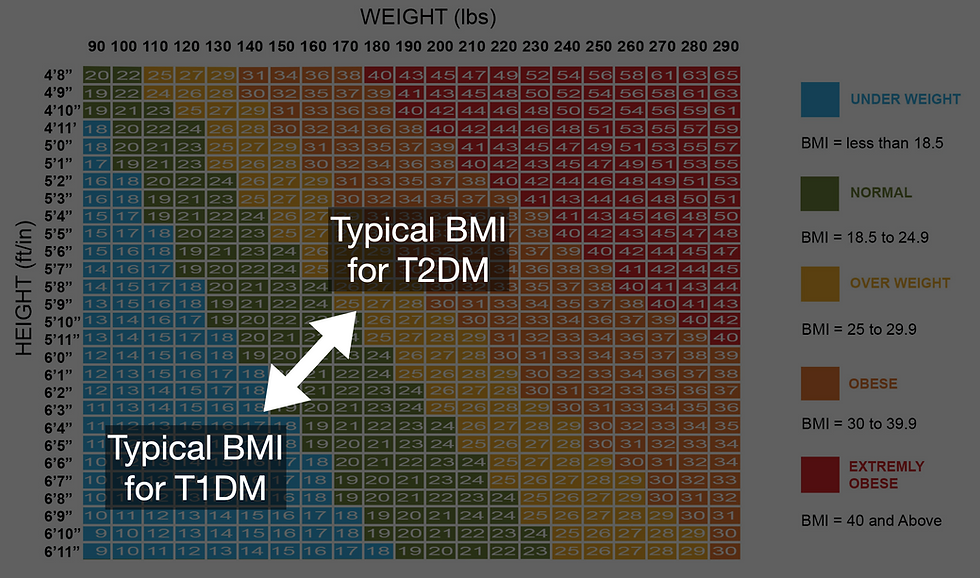
The glucose levels can get much more elevated in HHS than DKA as well. The DKA patient usually has a reading >250 mg/dL, but occasionally will have no hyperglycemia at all (euglycemic DKA). The HHS patient will routinely have a reading >600 mg/dL. Usually, when people tell you about the highest blood glucose they've ever seen, it's HHS. The highest I've seen was just over 1,400 mg/dL in a very sick and dehydrated HHS patient. But, of course, that wasn't what our glucometer said - it just said "HI". How come?
Glucometers have their limits for high and low, and it's a good idea to figure out what your glucometer has for limits. Just look at the make and model of the one you carry, and Google can usually get you where you need to go to figure it out. I've found that the average newer glucometer will read 'low' below 20 mg/dL, and 'high' above 500 or 600 mg/dL. This actually presents a logistical challenge for hospital-to-hospital transport, since our glucometers can't see readings above a certain number. Let me present you with a brief scenario.
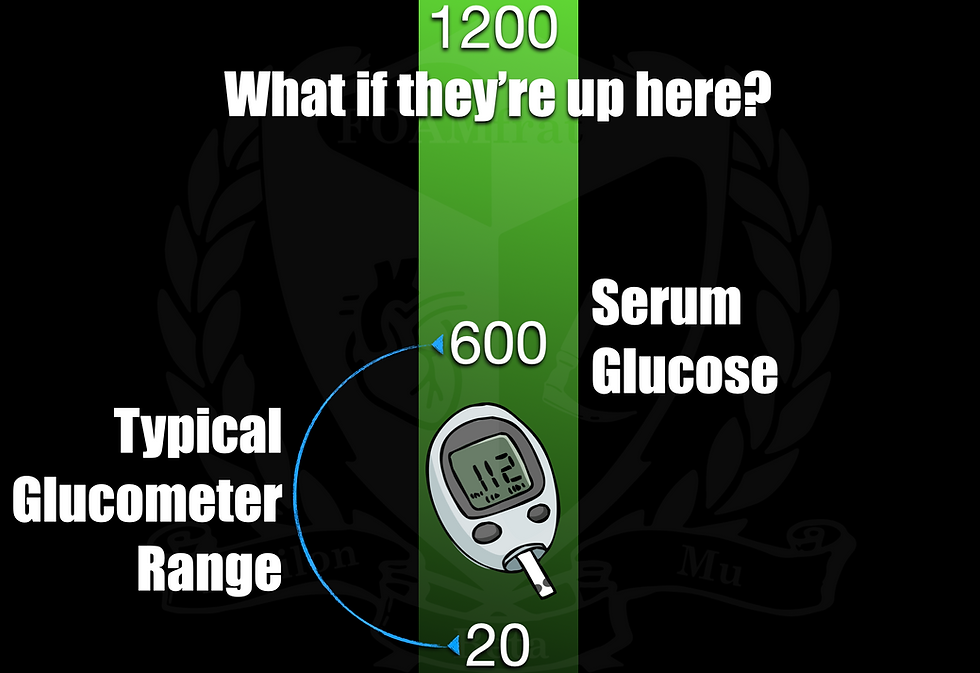
You arrive at the sending ED for an HHS (or DKA) patient with a BGL of 1,000 mg/dL. They have given the patient fluid replacement, managed electrolytes, and other checklist items mentioned above. They're now starting to administer insulin. But, remember how you can only lower glucose by ~75mg/dL per hour? How are you going to ensure that the glucose is coming down in a controlled manner if all your glucometer is reading is 'HIGH'? There isn't a perfect answer to this question until technology improves, but I'll tell you my personal opinion, without any real evidence to back it up.
In the DKA patient:
Assess how quickly the BGL has been coming down given the current infusion rate of insulin. If it's been very controlled, I'll probably leave the insulin where it is, or perhaps (depending on the patient) run a small amount of dextrose concurrently. That might seem stupid to run insulin and dextrose concurrently, but not if you understand what makes a DKA patient sick in the first place (it's about closing the anion gap - not lowing the glucose).
In the HHS patient:
I'll start off the same by assessing how quickly the blood glucose has been lowing and gauge my response from there. If it's been spot on, I'll probably just lower the infusion slightly for a cushion of safety and continue other treatments.
In both of these patients, the more severe dangers of cerebral edema should not come into play until the patient reaches a blood glucose level under about 250 or 300 mg/dL, or if you drop really quickly in general. If you're monitoring the glucose, you should see when it comes into range of your glucometer, and you've already assessed the rate that it's lowering, so you should be fine. However, that was all my personal opinion, don't take it as gospel.

Glucose readings are the first lab value that we're introduced to in school. We learn what to do if it's low, and then later on what to do if it's high. However, there's clearly more nuance to these blood glucose readings than we may initially think. Glucose is under hormonal and allosteric regulation of several processes that we should be very familiar with. As for actual patient treatment, for hypoglycemia, it's all about patient safety, understanding our concentrations of dextrose, as well as having a solid knowledge base on our catabolic hormone - glucagon. On the other side of treatment, when we're addressing hyperglycemia, it can get pretty complex. Ventilation, volume replacement, other electrolytes (like potassium), and one of our main anabolic hormones - insulin - come into play. I hope that this series of blogs and podcasts have helped you understand the metabolic panel on a deeper level and that they serve as a reference for you.
Thanks for reading!
(The video is up above for YouTube)
Be sure to check out our EMS refresher!
References
Chourpiliadis C, Mohiuddin SS. Biochemistry, Gluconeogenesis. [Updated 2021 Aug 4]. In: StatPearls [Internet]. Treasure Island (FL): StatPearls Publishing; 2021 Jan-. Available from: https://www.ncbi.nlm.nih.gov/books/NBK544346/
Berg JM, Tymoczko JL, Stryer L. Biochemistry. 5th edition. New York: W H Freeman; 2002. Section 16.1, Glycolysis Is an Energy-Conversion Pathway in Many Organisms. Available from: https://www.ncbi.nlm.nih.gov/books/NBK22593/
Jiang, G., & Zhang, B. B. (2003). Glucagon and regulation of glucose metabolism. American journal of physiology. Endocrinology and metabolism, 284(4), E671–E678. https://doi.org/10.1152/ajpendo.00492.2002
Tesfaye, N., & Seaquist, E. R. (2010). Neuroendocrine responses to hypoglycemia. Annals of the New York Academy of Sciences, 1212, 12–28. https://doi.org/10.1111/j.1749-6632.2010.05820.x
Delaney, T. M., Helvey, J. T., & Shiffermiller, J. F. (2020). A Case of Salicylate Toxicity Presenting with Acute Focal Neurologic Deficit in a 61-Year-Old Woman with a History of Stroke. The American journal of case reports, 21, e920016. https://doi.org/10.12659/AJCR.920016
Chow, E., & Troy, S. B. (2014). The differential diagnosis of hypoglycorrhachia in adult patients. The American journal of the medical sciences, 348(3), 186–190. https://doi.org/10.1097/MAJ.0000000000000217
Sako, A., Yasunaga, H., Matsui, H., Fushimi, K., Hamasaki, H., Katsuyama, H., Tsujimoto, T., Goto, A., & Yanai, H. (2017). Hospitalization with hypoglycemia in patients without diabetes mellitus: A retrospective study using a national inpatient database in Japan, 2008-2012. Medicine, 96(25), e7271. https://doi.org/10.1097/MD.0000000000007271
Paul E. Marik, in Critical Care Nephrology (Second Edition), 2009 Endocrinology of the Stress Response during Critical Illness https://www.sciencedirect.com/topics/medicine-and-dentistry/stress-hyperglycemia
Lizzo JM, Goyal A, Gupta V. Adult Diabetic Ketoacidosis. [Updated 2021 Jul 18]. In: StatPearls [Internet]. Treasure Island (FL): StatPearls Publishing; 2021 Jan-. Available from: https://www.ncbi.nlm.nih.gov/books/NBK560723/
Milanesi A, Weinreb JE. Hyperglycemic Hyperosmolar State. [Updated 2018 Aug 1]. In: Feingold KR, Anawalt B, Boyce A, et al., editors. Endotext [Internet]. South Dartmouth (MA): MDText.com, Inc.; 2000-. Available from: https://www.ncbi.nlm.nih.gov/books/NBK278976/